New materials research sees transformations at an atomic level
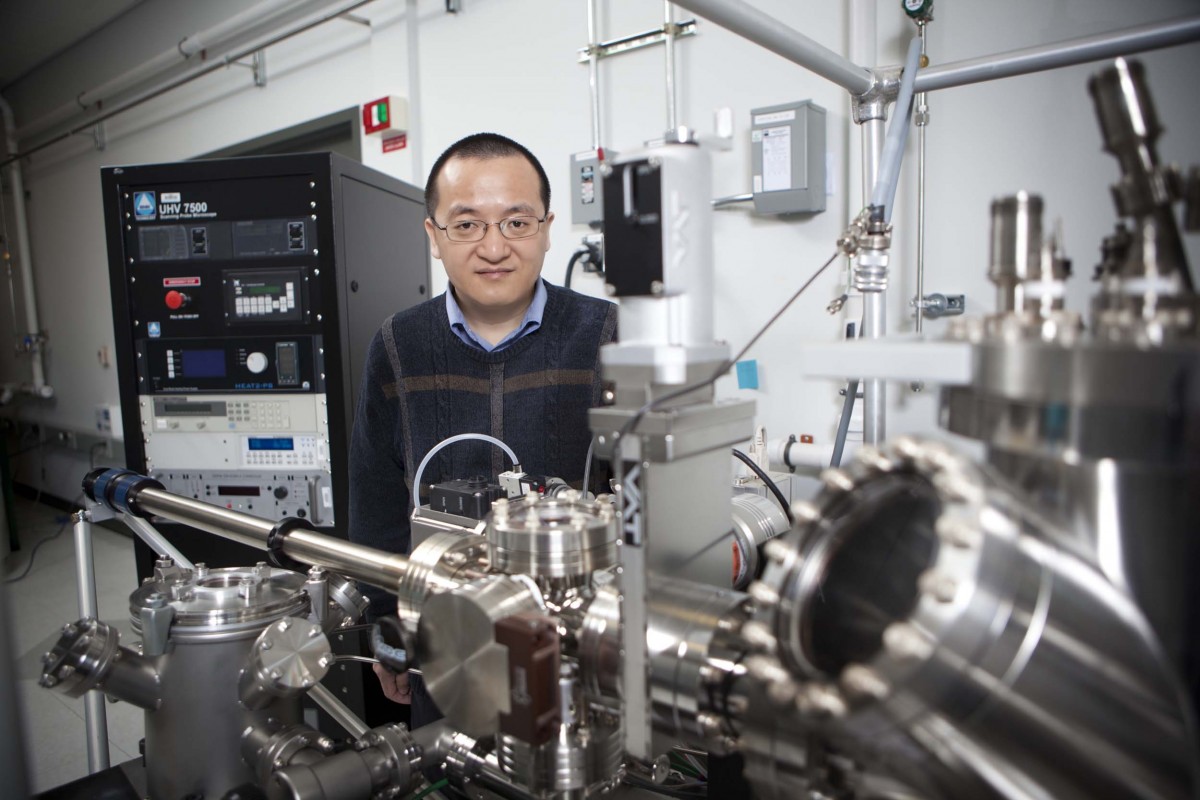
When manufacturing techniques turn metals, ceramics or composites into a technologically useful form, understanding the mechanism of the phase transformation process is essential to shape the behavior of those high-performance materials. Seeing those transformations in real time is difficult, however.
A new study in the journal Nature, led by Professor Guangwen Zhou from the Thomas J. Watson College of Engineering and Applied Science’s Department of Mechanical Engineering and the Materials Science program at Binghamton University, uses transmission electron microscopy (TEM) to peer into the oxide-to-metal transformation at the atomic level. Of particular interest are the mismatch dislocations that are ever-present at the interfaces in multiphase materials and play a key role in dictating structural and functional properties.
Zhou’s students Xianhu Sun and Dongxiang Wu are the first co-authors of the paper (“Dislocation-induced stop-and-go kinetics of interfacial transformations”). Sun recently finished his PhD thesis, and Wu is a PhD candidate. Other contributors are Lianfeng Zou, MS ’12, PhD ’17, now a professor at Yanshan University, and PhD candidate Xiaobo Chen; Professor Judith Yang, Visiting Research Assistant Professor Stephen House and postdoctoral researcher Meng Li from the University of Pittsburgh’s Swanson School of Engineering; and staff scientist Dmitri Zakharov from the Center for Functional Nanomaterials, a U.S. Department of Energy (DOE) Office of Science User Facility at Brookhaven National Lab.
Using the advanced technique, Zhou said, “manufacturers may be able to control the microstructure and properties of current materials and design new types of materials. There is some practical importance for this research, but there’s a fundamental significance as well.”
The experiments tested the transformation of copper oxide to copper. Directly observing such an interface transformation at the atomic scale is challenging because it requires a capability not only to access the buried interface but also to apply chemical and thermal stimuli to drive the transformation.
By using environmental TEM techniques capable of introducing hydrogen gas into the microscope to drive the oxide reduction while simultaneously performing TEM imaging, the research team was able to atomically monitor the interfacial reaction. Surprisingly, the researchers observed that the transformation from copper oxide to copper occurs in an intermittent manner because it is temporarily stopped by mismatch dislocations, a behavior similar to a stop-and-go process regulated by traffic lights.
“This is unexpected, because the common sense accepted by the materials research community is that interface dislocations are the locations to facilitate the transformation rather than to delay it,” Zhou said.
To understand what was at work, Wu developed computer codes to explain what they were witnessing in experiments. This back-and-forth process between experiments and computer modeling helped the team understand how misfit dislocations control the long-range transport of atoms needed for the phase transformation.
“This looping, iterative process between experiments and computer modeling, both at the atomic level, is an exciting aspect for materials research,” Zhou said.
The fundamental information could prove useful in designing new types of multiphase materials and controlling their microstructure, which can be used in diverse applications such as load-bearing structural materials, electronic fabrication and catalytic reactions for clean energy production and environmental sustainability.
After collecting initial data at Binghamton, Sun and the research team repeated the experiments on equipment at Pitt and Brookhaven, which have different capabilities.
“This is a collaborative work. Without the facilitates at Brookhaven Lab and the University of Pittsburgh, we cannot see what we need to see,” Sun said. “Also, in the late stages of my analysis data, I talked through the results with Judy, Meng and Dmitri many times. I remember when we finished the first draft and sent the manuscript to Dmitri, he told me that maybe we should include some equations to confirm our observed results, and he sent some relevant literature. So now we can show those calculations agree with our experimental results.”
Yang also called the research “a really nice partnership” that brought together the best elements of Binghamton, Pitt and Brookhaven.
“The ability to use forefront tools is one of the things that underpins new science, as exemplified here,” she said. “Brookhaven has an exceptional microscope that can take environmental stress at higher pressures than the one we have at the University of Pittsburgh, and it has higher analytical capability. But the University of Pittsburgh one is a good high-resolution transmission electron microscope that can accept gas, it’s a more robust microscope. There’s also more research time available.”
She used an analogy to explain why seeing chemical reactions happen in real time is important: “When you buy fish and it’s packaged, there’s only so much you can understand about that fish as opposed to seeing the fish in a real environment.”
Because the DOE national labs can offer state-of-the-art instruments and top-caliber expertise that complements what’s available at universities and high-tech industry, they can help researchers — especially those early in their careers — take their work to the next level, in most cases for free.
Zakharov said he is glad to have played a part in this materials research: “The power of the technique is that it’s a direct method to see all these dislocations and phase transformations. You can control the reaction, and you can go back and forth to observe how those dislocations in the interfaces behave. There is not any other technique with such a direct observation.”
Sun — who now works at the Lawrence Berkeley National Laboratory, also a DOE National Lab — is happy to have this research finally published.
“I started to analyze this data in March 2018, so it’s taken almost five years to finish this work,” he said. “It’s challenging, but it’s worth it.”
This work was supported by the U.S. Department of Energy’s Basic Energy Science. The research used the Electron Microscopy Facility of the Center for Functional Nanomaterials and the Scientific Data and Computing Center at Brookhaven National Laboratory, which is supported by the U.S. Department of Energy’s Office of Basic Energy Sciences. This research also used the Environmental TEM at the University of Pittsburgh with support through a National Science Foundation Major Research Instrumentation award.