Our Research Highights
Energy Harvesting from Triboelectric Effect for Smart Knee Implants
A total knee replacement (TKR) restores range of motion and provides pain relief primarily for patients suffering from osteoarthritis. While durability has improved significantly, these implants can still fail prematurely, especially because of improper soft tissue balancing or overloading if the patient exceeds exercise limitations. These failures could be mitigated by intra- and post-operative load sensing, respectively. The development of a sensor system capable of measuring and reporting forces transmitted through TKR is one of the next logical evolutions of these implants. While some sensor systems have been proposed, they all require external power provided via induction coils or an internal battery that will eventually become depleted. We propose that energy can be harvested from the loads passing through the joint during the activities of daily living using the triboelectric effect. The triboelectric effect is a newly discovered transduction mechanism for converting mechanical energy into electrical energy. It has a higher power density than other mechanisms such as electromagnetic and piezoelectric transduction, and therefore allows a smaller overall form factor. This means the sensor can be installed between the tibial tray and UHMWPE bearing component of any TKR without any modifications.
The objective of this research is to create a self-powered load measurement system
for TKR. Studies will focus on i) developing a model that can accurately predict the
output power of the energy harvester, ii) manufacturing a physical prototype as a
proof-of-concept, and iii) comprehensive testing. The model will enable optimizing
the energy harvester design to maximize power generation. We will integrate the energy
harvester into a low-power sensing and telemetry system capable of transmitting the
measured data wirelessly to an external receiver. The integrated system will be tested
by a joint simulator under typical knee loading. The sensor accuracy in measuring
the load will be quantified. Load imbalances such as improper soft tissue tensions
or implant component misalignment will be simulated and the sensor's capability in
detecting these issues will be determined. Because the sensor will interface directly
with other implant components, we will also perform long-term durability studies to
rule out any potential detrimental effects on implant longevity as a result of introducing
our sensor. Once developed and tested, our sensor will offer an option for continuous
monitoring of TKR health. This study is funded by the National Institute of Health.
You can read our published studies in Smart Materials and Structures, IEEE Sensors Journal, IEEE Transactions on Mechatronics, and ASME Journal of Biomechanical Engineering.
Read press release on our work here.
MEMS Actuators and Sensors using electrostatic levitation
MEMS actuators capable of large out-of-plane displacements and large rotations are desired for optical applications such as optical coherence tomography, endoscopy and interferometry. Our main method of actuation is electrostatic actuation due to the low power consumption and easy fabrication.
Among different electrostatic actuation schemes, repulsive (electrostatic levitation) force mechanism is an effective method as it avoids pull-in instability and enables large range of motion. Because of this capability, repulsive force scheme has a great potential for developing capacitive sensors used in accelerometers, gyroscopes, microphones, and filters. Employing this new sensing scheme, high resolution and unprecedented sensitivity can be achieved, which is the subject of our recent project. The project is titled "A New Approach to Capacitive Sensing: Repulsive Sensors" and is funded by the National Science Foundation.
We simulate and characterize these actuators/sensors to predict their responses to different excitation levels. These simulation studies are used to optimize their design parameters for the best performance.
We created novel devices that make use of electrostatic levitation (repulsive) actuators including Pull-in free MEMS microphones, large-stroke accelerometers, robust MEMS switches, Pressure switches, T-beam Resonators, and band-pass filters.
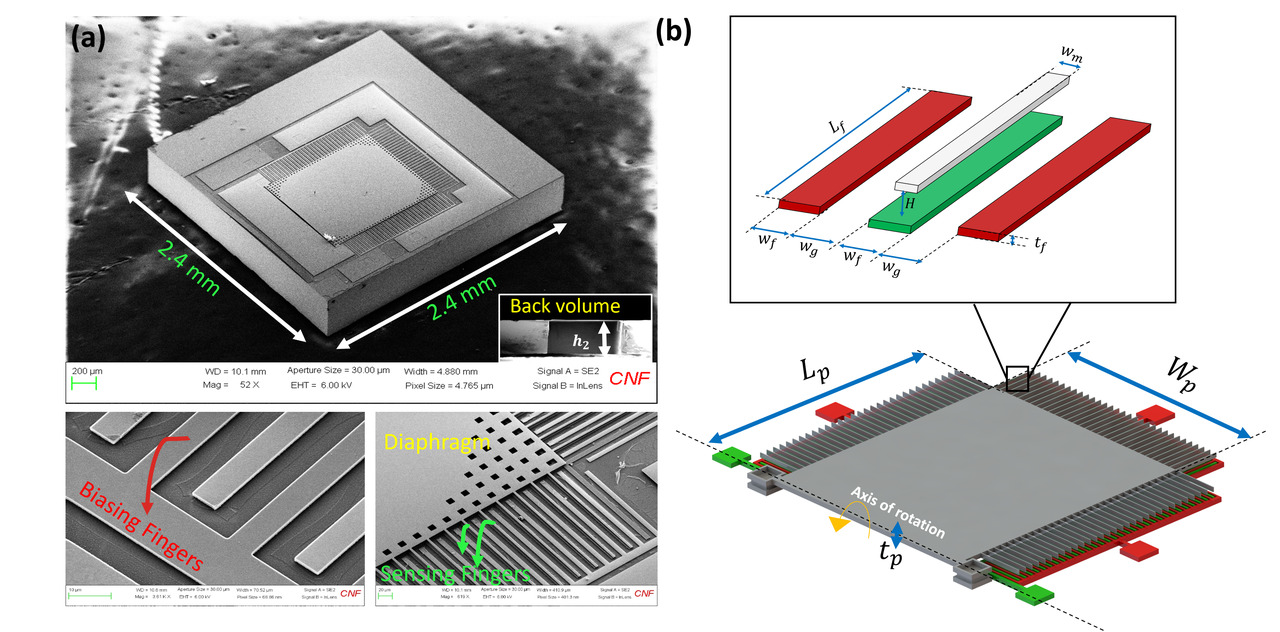
Figure: Silicon wafer that contains many MEMS microphones using repulsive (levitation) sensors. These devices are widely used in cell phones and portable electronics.
Figure: (a) a MEMS pressure switch using electrostatic levitation and parallel-plate electrodes; (b) Pressure vs pull-in voltage at various pressures. The combined system enables detecting a threshold pressure by sudden closing of the MEMS switch. See the published work here.
Autonomous Micro Switch Operated by Mechanical Shock
This project is funded by the National Science Foundation. It aims to create a self-operated mechanical shock switch system. The proposed mechanism merges two transducers, one which converts mechanical shock to voltage and another which converts voltage to motion to open a micro-switch. The result is a self-operated sensor directly operated by mechanical shock when the shock goes beyond a threshold. Currently, shock sensors such as those in air-bag deployment devices need a processing unit to engage the device, which requires a complicated control system having risks of software and component failures. The proposed system eliminates the need for signal processing units by synergistically connecting two transducers that overcome the limitations of each system. The result is a system that reduces the risk of component failure and increases reliability. The proposed system has promising applications in earthquake detection systems that need to trigger an immediate response to significant seismic activity such as shutting off power lines and sounding alarms. Wider applications include safety switches in the automotive industry and shipment monitors.
One of our papers published on this project won the ASMS branch of ASME 2020 award of Materials and Systems. The award is very competitive and high prestigious. Out work is titled "Pairing electrostatic levitation with triboelectric transduction for high-performance self-powered MEMS sensors and actuators" published in Applied Physics Letters. Other recent studies include Autonomous shock sensing that was published in Smart Materials and Structures.
We are excited about making the next generation of Triboelectric generators compatible with the MEMS Technology fabrication. This capability opens doors to creation of billions of sensors and switches that are event powered. Our recent study is under review at the Nano Energy journal.
Figure: Schematic of the combined system of generator and MEMS switch. The MEMS switch opens when the generator receives an impact beyond a threshold, so the combined system acts as a self-powered shock sensor.
Figure: 3D Model of the tribeoelctric generator where the top layer consists of a patterned conductor layer suspended by springs above Polyimide on a conductor layer.
Figure: SEM image of the top layer of the MEMS triboelectric nanogenerator (left) release holes, (right) pins.
Energy harvesting from Nonlinear Mechanical Vibration
Mechanical vibration present in the environment and transportation vehicles is an
abundant source of energy that can be used to operate remote sensors to detect early
signs of failure. We are developing compact and high performance nonlinear resonators
that can efficiently harvest energy from broadband ambient mechanical vibration below
100 Hz to operate self-powered sensors. Using nonlinear resonators with piezoelectric
and triboelectric transducers, milliwatts of power can be generated that is sufficient
for transmitting essential data. The nonlinear resonators are electromechanical systems
that exploit bi-stability, impact, internal resonance and parametric resonance to
increase the frequency bandwidth of energy harvesting. The increased bandwidth increases
the energy conversion efficiency as the frequency spectrum is spread over a larger
range of frequency resembling that of the ambient vibrations. Exciting with a random
vibration signal, we are evaluating the output power. The product of this research
can be used for autonomous wireless sensors in remote places.
Figure: Energy harvesting mechanisms exploiting nonlinear dynamic characteristics to increase the frequency bandwidth
One of our work on nonlinear energy harvesting won the 2020 BEST ENERGY HARVESTING AWARD governed by the energy harvesting technical committee as part of the ASMS branch of the ASME. The paper citation info is "W. Yang, S. Towfighian (2019), A Parametric Resonator with Low Threshold Excitation for Vibration Energy Harvesting, Journal of Sound and Vibration, Vol. 446, pp. 129-143".